This article is part of “Innovations In: Sickle Cell Disease,” an editorially independent special report that was produced with financial support from Vertex Pharmaceuticals.
Sickle cell disease might seem simple enough: it’s caused by a single mutation in a single gene. But the way it affects patients is remarkably complex—so complex that 70 years after that mutation was discovered, treatment remains difficult. In people with healthy red blood cells, hemoglobin proteins carry oxygen as the cells circulate throughout the body. In patients with sickle cell, the namesake cells form when mutant hemoglobin proteins clump together into fibers, deforming red blood cells’ usual saucer shape into a curved sickle, which prevents them from effectively delivering oxygen to tissues. These sickle cells can get stuck inside blood vessels, interrupting blood flow and often prompting intense pain and a crisis so severe it sends people to the hospital and puts them at risk of cardiac failure and other life-threatening issues. Over the long term, disrupted blood flow, oxidative stress and inflammation combine to cause strokes and permanent damage to blood vessels and other organs.
The disease strikes in so many ways that researchers are attacking the problem from multiple angles: at the source by preventing bone marrow from producing mutant red blood cells in the first place; at the cellular level by modifying the misshapen red blood cells’ metabolism and protein structure; and at the immune level to prevent long-term organ damage. “The problem is (it’s) all connected,” says Marilyn Telen, a hematologist at Duke University. “So where do you build the dam?”
On supporting science journalism
If you’re enjoying this article, consider supporting our award-winning journalism by subscribing. By purchasing a subscription you are helping to ensure the future of impactful stories about the discoveries and ideas shaping our world today.
The ideal place to halt the disease is at its source: if bone marrow stem cells are generating the faulty red blood cells, replace them with ones that produce healthy cells. The most established method for this approach is a bone marrow transplant from a matched donor. The procedure requires eliminating the patient’s original bone marrow cells pretransplant, using a course of strong chemotherapy, to create a niche for the introduced cells to grow in. The treatment can cause infertility and necessitates a long course of immunosuppressive drugs—often continued for years—to prevent the body from rejecting its transplant.
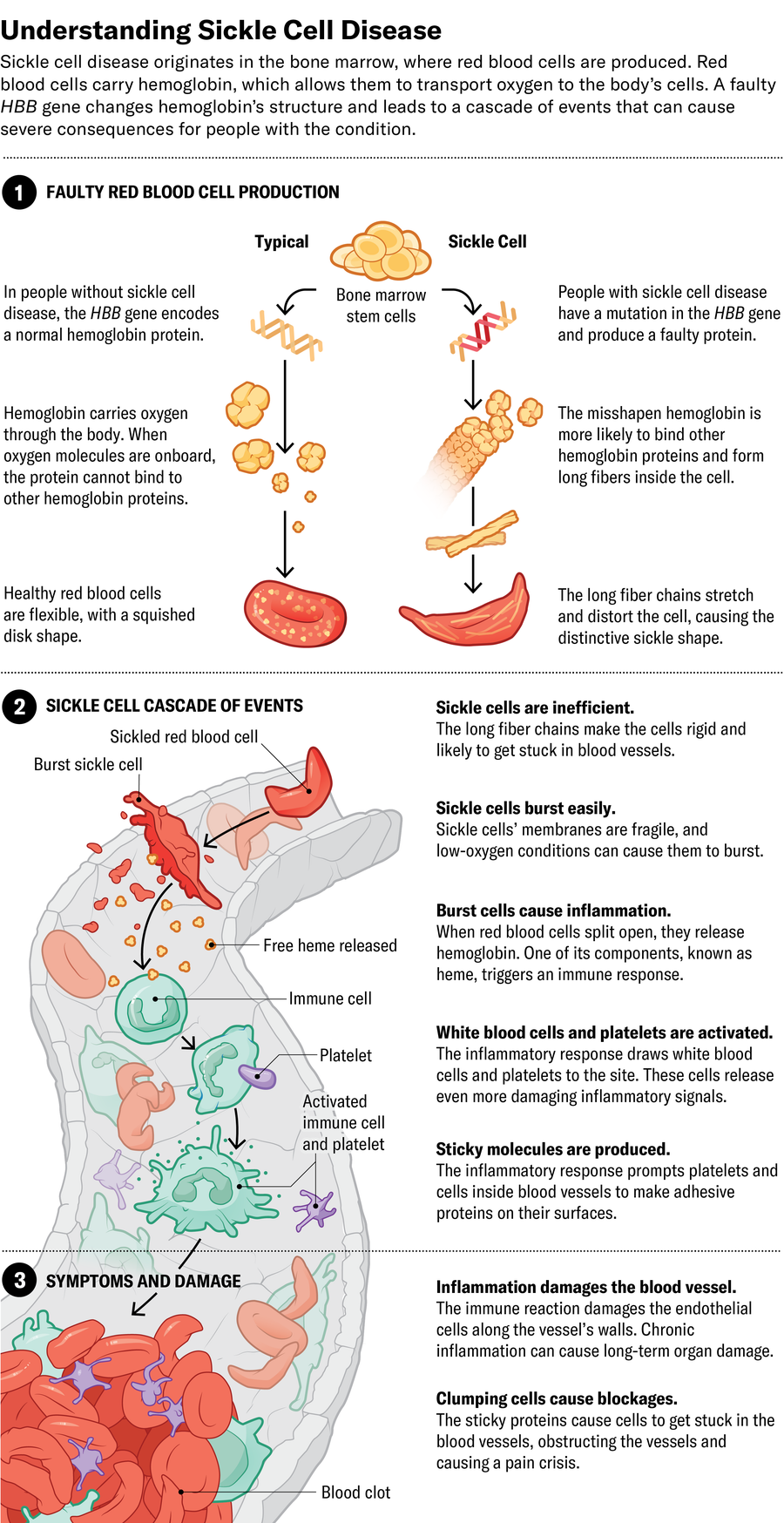
Now Medical Studios; Source: Kelly Rose, chief scientific officer at American Society of Hematology (consultant)
To try to improve on the success of bone marrow transplants, some companies have created gene-editing approaches that eliminate the need for immunosuppressive drugs. They remove patients’ stem cells, modify the cells’ DNA in a laboratory to prompt the production of healthy hemoglobin, then reintroduce them to the patient. A number of these gene-modifying approaches have already made their way to clinical trials or been approved by the U.S. Food and Drug Administration.
The form of hemoglobin that babies make at birth, called fetal hemoglobin (HbF), isn’t affected by the sickle mutation. Typically HbF is mostly replaced by the adult version of hemoglobin within a few months after birth, leading several companies to focus on increasing the amount of HbF in the bloodstream. In 2023 the FDA approved Vertex Pharmaceuticals’s exa-cel, which uses CRISPR-Cas9 gene editing to deactivate the gene that prevents production of HbF. The result is a higher fetal-to-adult hemoglobin ratio.
Editas Medicine is also enhancing HbF production, using a different CRISPR-related enzyme called Cas12a to do the editing. Whereas Cas9 edits DNA by breaking both strands of the double helix in the same place, Cas12a breaks them at different positions and results in more consistent edits. Beam Therapeutics is pursuing a similar method, using another form of CRISPR to swap single nucleotides in the genetic code that enhance HbF production. Both therapies are still in clinical trials.
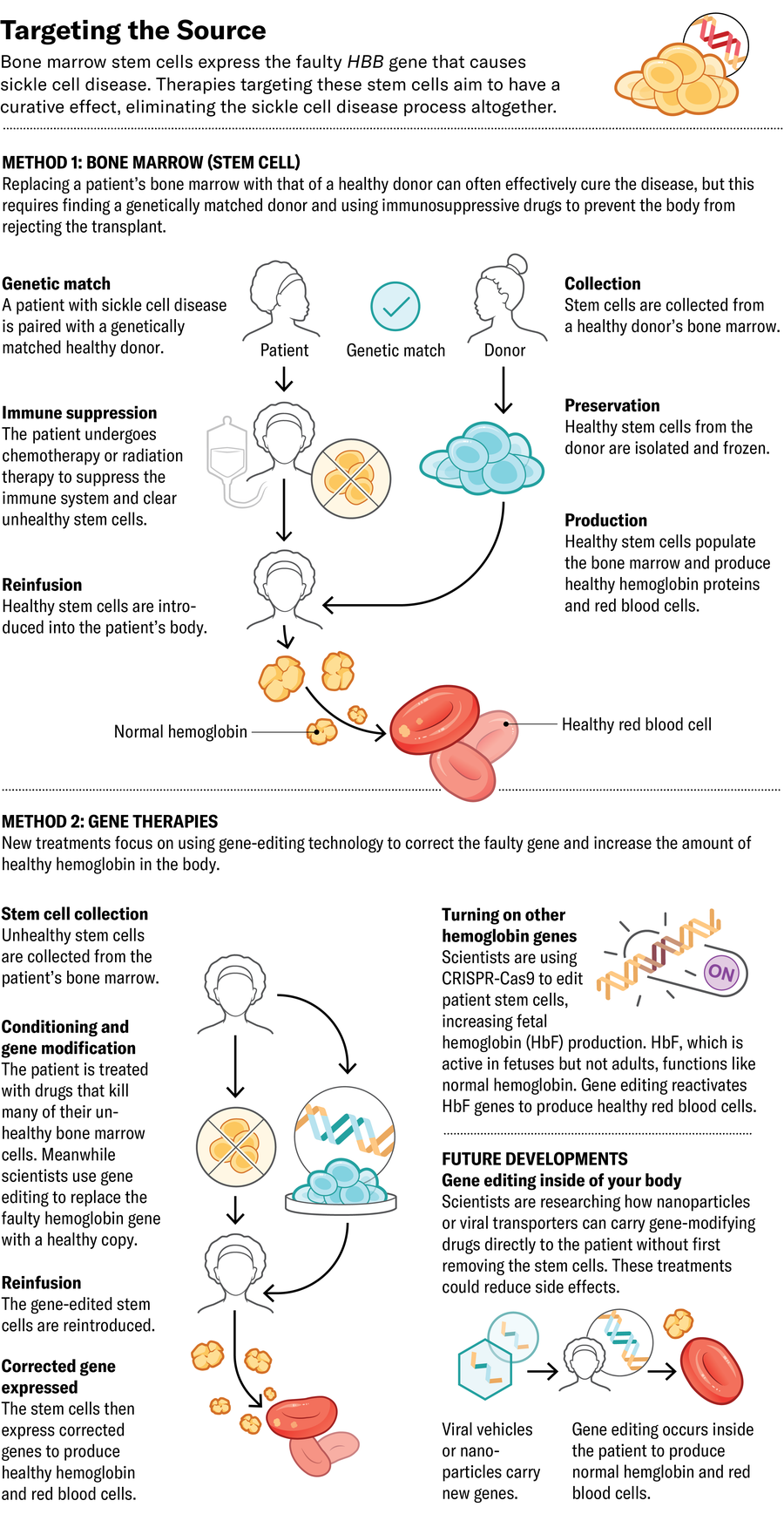
Now Medical Studios; Source: Kelly Rose, chief scientific officer at American Society of Hematology (consultant)
A different strategy is to insert a more resilient version of the gene encoding adult hemoglobin rather than increasing HbF. Bluebird bio’s lovo-cel, which also received FDA approval last year, uses a more classical gene therapy approach. Rather than editing a patient’s DNA, it uses a modified virus to deliver a healthy, enhanced copy of the adult hemoglobin gene into bone marrow stem cells, where it integrates into the patient’s DNA.
These new technologies, says Alexis Thompson, a pediatric hematologist at Children’s Hospital of Philadelphia, are “an improvement over the many years and decades when we had very little to offer families.”
A future approach might let doctors edit patients’ bone marrow without removing it from the body. In a study published last year in Science, researchers described the development of nanoparticles that carry messenger RNA (mRNA) into stem cells in the bone marrow. When they used the particles in cells from people with sickle cell disease, the editing system encoded by the mRNA successfully modified the hemoglobin gene in those cells’ genomes to produce more healthy hemoglobin protein.
It may be as many as 10 to 20 years before such in vivo technology is ready for large-scale human trials, Telen says. “We know the technology we need. We know a lot of the pieces. We just haven’t been able to get it to all work together.”
In the meantime current gene therapies remain expensive and challenging to scale up because each patient’s batch of cells has to be edited individually. The treatments are out of reach for most people with the disease, particularly in sub-Saharan Africa, where 75 percent of all sickle cell cases occur.
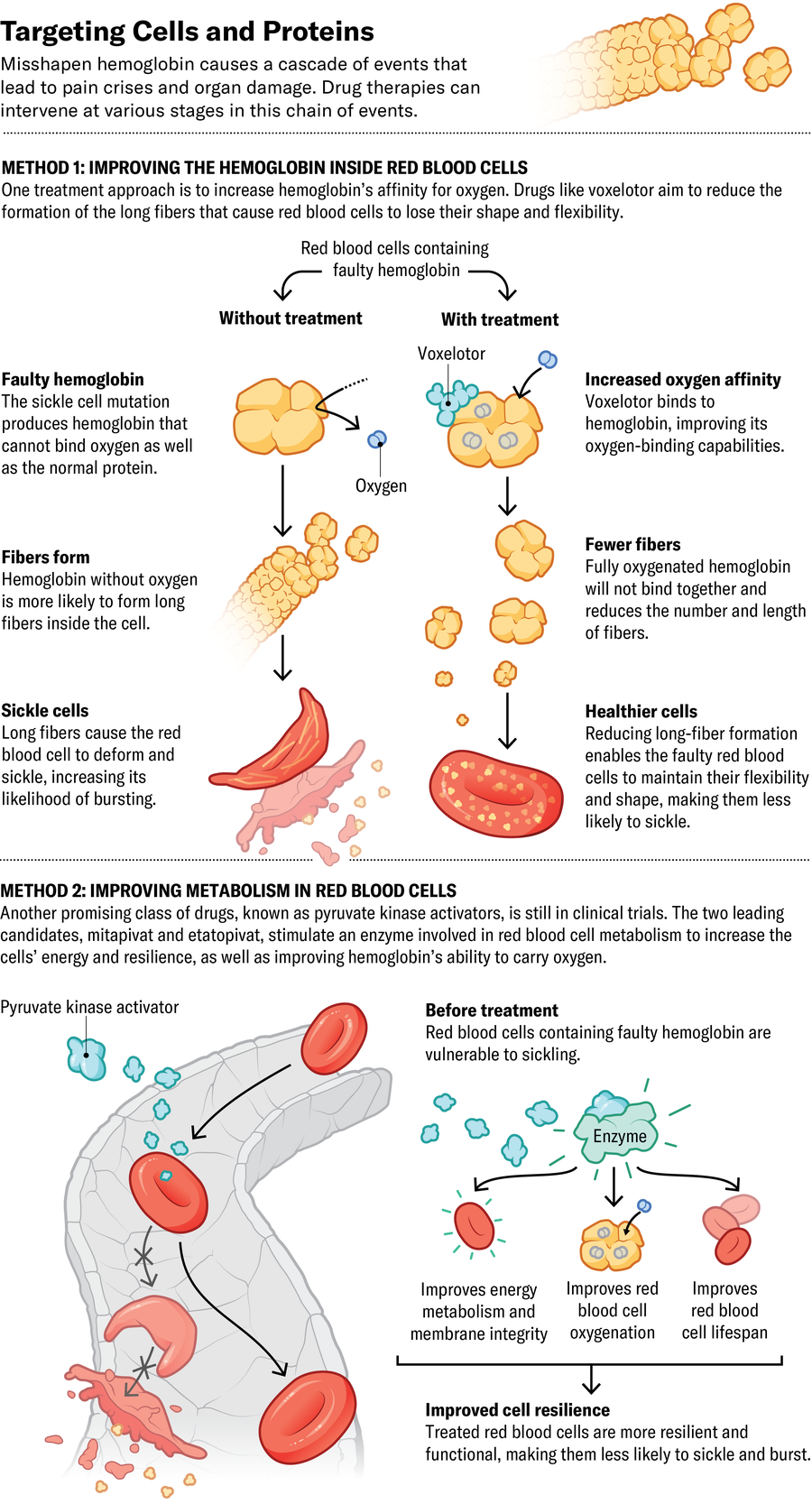
Now Medical Studios; Source: Kelly Rose, chief scientific officer at American Society of Hematology (consultant)
All of this means that, for most people, the most accessible curative treatment is bone marrow transplantation from a donor with specific, genetically matched cellular markers. (Such matches are often but not always found among close family members.) And now a technique initially developed for treating blood cancers could make more transplants possible by expanding the donor pool. A strategy that includes a brief course of two chemotherapy drugs—thiotepa and cyclophosphamide—suppresses the immune systems of bone marrow recipients enough to allow them to receive transplants from almost any donor who shares half of their DNA, such as a parent, cousin or sibling without sickle cell disease.
In a 54-person trial, researchers found that around 90 percent of sickle cell patients treated with this technique who received transplants from half-matched donors went at least two years without rejection. Some patients appeared completely cured, says hematologist Adetola Kassim of Vanderbilt University, who led the study. And unlike gene therapy, this “haploidentical transplant” requires only a brief and far less toxic course of chemotherapy. Kassim says this approach may be a more affordable option: “The technique we use is exportable and scalable.”
When bone marrow transplants are out of reach, treatments that act further downstream can address physiological issues, such as hemoglobin clumping, and ameliorate symptoms. “The argument could be made that the vast majority of sickle cell patients in the world are unlikely to get curative treatment,” Telen says. “So it behooves us to bring other drugs onto the market to improve their lives.”
The FDA has approved three sickle cell drugs in recent years, and dozens more are in the pipeline. The first new drug, approved in 2017, was Emmaus Medicine’s l-glutamine, an antioxidant that helps to maintain red blood cells’ round shape. In a clinical trial, patients consuming l-glutamine powder mixed into food or drinks had 25 percent fewer pain crises than those in the placebo group.
Pfizer’s voxelotor and osivelotor, the latter of which is in clinical trials, both bind directly to hemoglobin and increase its ability to hang on to oxygen. This action also prevents the protein from clumping and causing red blood cells to lose their shape. The FDA and European Commission approved voxelotor in 2019 and 2022, respectively, after data showed it could raise hemoglobin levels and protect against severe anemia. “When it works, it’s amazing. It’s somewhat of a miracle drug,” says Jeffrey Glassberg, an emergency medicine physician specializing in sickle cell at the Icahn School of Medicine at Mount Sinai in New York City. But some patients don’t respond, and a phase 3 clinical trial suggests it does not reduce the number of pain crises.
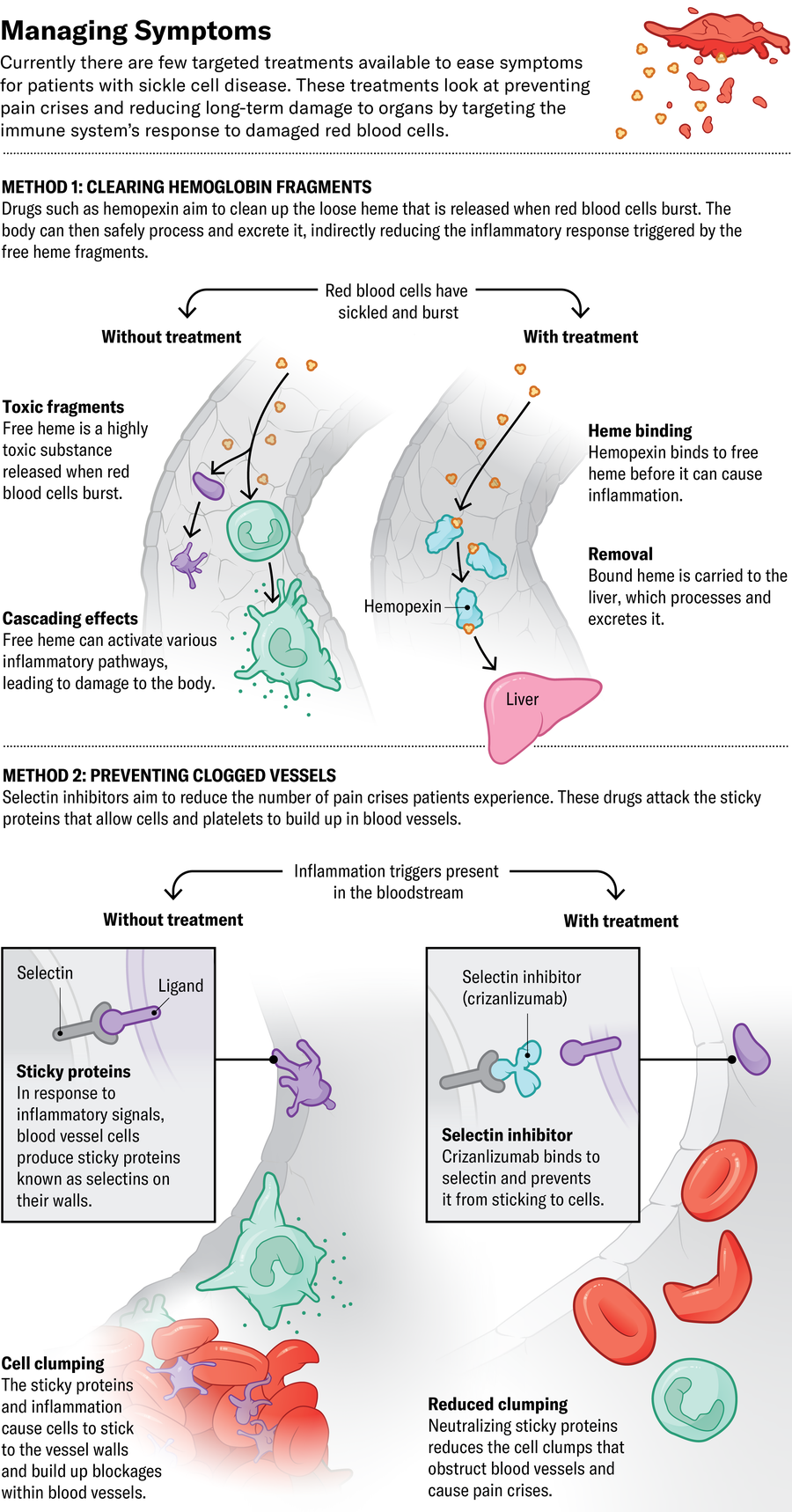
Now Medical Studios; Source: Kelly Rose, chief scientific officer at American Society of Hematology (consultant)
Another promising class of drugs, known as pyruvate kinase activators, stimulate an enzyme involved in cellular metabolism to both increase cells’ energy and improve hemoglobin’s ability to carry oxygen. The two leading candidates, Agios Pharmaceuticals’s mitapivat and Novo Nordisk’s etavopivat, are in clinical trials.
Physicians still have few choices for helping someone in the throes of an attack. Typically the only available options are pain medication and intravenous fluids. But new drugs that target something other than red blood cells might help with acute crises and reduce the immune reactions that contribute to pain and organ damage over time.
The third drug recently approved by the FDA, Novartis’s crizanlizumab, attacks sticky proteins called selectins on blood vessels and platelet cells. Blocking those proteins prevents red blood cells from clumping together inside the vessels, supposedly preventing pain crises. But it may not be quite that simple. The European Medicines Agency revoked its approval of crizanlizumab in 2023 after a review committee found it didn’t seem to reduce the number of crises people experienced. Glassberg thinks the drug is still useful—his unpublished research suggests that crizanlizumab and similar drugs currently in clinical trials could reduce long-term organ damage and chronic kidney disease.
Other approaches aim to diminish immune system activity. CSL Behring is conducting clinical trials of hemopexin, a drug that cleans up hemoglobin that has broken free of red blood cells. Loose hemoglobin causes cells to clump in blood vessels and contributes to inflammation, leading to long-term organ damage. Researchers elsewhere are investigating whether tamping the activity of certain immune-signaling molecules known as complement proteins might prevent the same inflammation-based injury.
Now Glassberg and others are trying to determine which of these drugs work best and whether certain combinations might work synergistically. He is currently running a trial at Mount Sinai called REAL Answers, in which 1,200 patients will receive the newly FDA-approved drugs, as well as an older one called hydroxyurea, alone or in combinations. Glassberg’s team is assessing their effects on pain crises, monitoring for signs of organ injury and searching for genetic markers that could predict how well someone will respond.
It’s too soon to know how most of these new therapies will fit into patient care. They might be most effective when started early in life. The drugs have not yet been extensively tested for safety and efficacy in children, but such an approach might prevent organ damage that can start early and then worsen over time.