Physicists seem to be obsessed with cats. James Clerk Maxwell, the father of electrodynamics, studied falling felines to investigate how they turned as they fell. Many physics teachers have used a cat’s fur and a hard rubber rod to explain the phenomenon of frictional electricity. And Erwin Schrödinger famously illustrated the strangeness of quantum physics with a thought experiment involving a cat that is neither dead nor alive.
So it hardly seems surprising that physicists turned to felines once again to name a newly discovered quantum phenomenon in a paper published in the New Journal of Physics in 2013. Their three-sentence study abstract reads, “In this paper we present a quantum Cheshire Cat. In a pre- and post-selected experiment we find the Cat in one place, and its grin in another. The Cat is a photon, while the grin is its circular polarization.”
The newfound phenomenon was one in which certain particle features take a different path from their particle—much like the smile of the Cheshire Cat in Alice’s Adventures in Wonderland, written by Lewis Carroll—a pen name of mathematician Charles Lutwidge Dodgson—and published in 1865. To date, several experiments have demonstrated this curious quantum effect. But the idea has also drawn significant skepticism. Critics are less concerned about the theoretical calculations or experimental rigor than they are about the interpretation of the evidence. “It seems a bit bold to me to talk about disembodied transmission,” says physicist Holger Hofmann of Hiroshima University in Japan. “Instead we should revise our idea of particles.”
On supporting science journalism
If you’re enjoying this article, consider supporting our award-winning journalism by subscribing. By purchasing a subscription you are helping to ensure the future of impactful stories about the discoveries and ideas shaping our world today.
Recently researchers led by Yakir Aharonov of Chapman University took the debate to the next level. Aharonov was a co-author of the first paper to propose the quantum Cheshire effect. Now, on the preprint server arXiv.org, he and his colleagues have posted a description of theoretical work that they believe demonstrates that quantum properties can move without any particles at all—like a disembodied grin flitting through the world and influencing its surroundings—in ways that bypass the critical concerns raised in the past.
A Grin without a Cat
Aharonov and his colleagues first encountered their quantum Cheshire cat several years ago as they were pondering one of the most fundamental principles of quantum mechanics: nothing can be predicted unambiguously. Unlike classical physics, the same quantum mechanical experiment can have different outcomes under exactly the same conditions. It is therefore impossible to predict the exact outcome of a single experiment—only its outcome with a certain probability. “Nobody understands quantum mechanics. It’s so counterintuitive. We know its laws, but we are always surprised,” says Sandu Popescu, a physicist at the University of Bristol in England, who collaborated with Aharonov on the 2013 paper and the new preprint.
But Aharonov was not satisfied with this uncertainty. So, since the 1980s, he has been exploring ways to investigate fundamental processes despite the probability-based nature of quantum mechanics. Aharonov—now age 92—employs an approach that involves intensively repeating an experiment, grouping results and then examining what came out before and after the experiment and relating these events to each other. “To do this, you have to understand the flow of time in quantum mechanics,” Popescu explains. “We developed a completely new method to combine information from measurements before and after the experiment.”
The researchers have stumbled across several surprises with this method—including their theoretical Cheshire cat. Their idea sounds simple at first: send particles through an optical tool called an interferometer, which causes each particle to move through one of two paths that ultimately merge again at the end. If the setup and measurements were carried out skillfully, Aharonov and his colleagues theorized, it could be shown that the particle traveled a path in the interferometer that differed from the path of its polarization. In other words, they claimed the property of the particle could be measured on one path even though the particle itself took the other—as if the grin and the cat had come apart.
Inspired by this theory, a team led by Tobias Denkmayr, then at the Vienna University of Technology, implemented the experiment with neutrons in a study published in 2014. The team showed that the neutral particles inside an interferometer followed a different path from that of their spin, a quantum mechanical property of particles similar to angular momentum: Denkmayr and his colleagues had indeed found evidence of the Cheshire cat theory. Two years later researchers led by Maximilian Schlosshauer of the University of Portland successfully implemented the same experiment with photons. The scientists saw evidence that the light particles took a different path in the interferometer than their polarization did.
Weak Measurements and Illusions
But not everyone is convinced. “Such a separation makes no sense at all. The location of a particle is itself a property of the particle,” Hofmann says. “It would be more accurate to talk about an unusual correlation between location and polarization.” Last November Hofmann and his colleagues provided an alternative explanation based on widely known quantum mechanical effects.
And in another interpretation of the Cheshire cat results, Pablo Saldanha of the Federal University of Minas Gerais in Brazil and his colleagues argue that the findings can be explained with wave-particle duality. “If you take a different view, there are no paradoxes,” Saldanha says, “but all results can be explained with traditional quantum mechanics as simple interference effects.”
Much of the controversy surrounds the way in which particles’ properties and positions are detected in these experiments. Disturbing a particle could alter its quantum mechanical properties. For that reason, the photons or neutrons cannot be recorded inside the interferometer using an ordinary detector. Instead scientists must resort to a principle of weak measurement developed by Aharonov in 1988. A weak measurement makes it possible to scan a particle very lightly without destroying its quantum state. This comes at a price, however: the weak measurement result is extremely inaccurate. (Thus, these experiments must be repeated many times over, to compensate for the fact that each individual measurement is highly uncertain.)
In the quantum Cheshire cat experiments, a weak measurement is made along a path in the interferometer, the paths then merge, and the emerging particles are measured with an ordinary detector. Along one path of the interferometer, a weak measurement of the particle’s position can be taken and, along the other, its spin. Using detectors, physicists can more definitively characterize the particles that traveled through the interferometer and potentially reconstruct what occurred during the particle’s journey. For example, only certain particles will appear in certain detectors, helping the physicists piece together which path their neutron or photon previously took. According to Aharonov, Popescu and their colleagues, the Cheshire cat experiments ultimately reveal that the particle’s position can be confirmed on one path even as its polarization or spin was measured on the other.
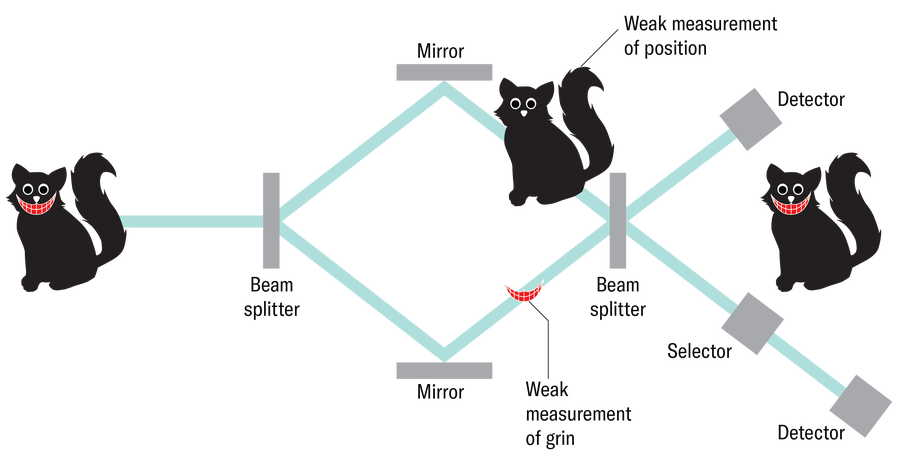
Saldanha and his co-authors assert that it is impossible to make claims about quantum systems in the past given their measurements in the present. In other words, the photons and neutrons measured in the final detectors cannot tell us much about their previous trajectory. Instead the wave functions of particles passing through the paths of the interferometer could overlap, which would make it impossible to trace which path a particle had taken. “Ultimately, the paradoxical behaviors are related to the wave-particle duality,” Saldanha says. But in the papers that report evidence of the quantum Cheshire cat, he asserts, the findings “are processed in a sophisticated way that obscures this simpler interpretation.”
Hofmann, meanwhile, has stressed that the results will differ if you measure the system in a different way. This phenomenon is well-known in quantum physics: if, for example, you first measure the speed of a particle and then its position, the result can be different than it would be if you first measured the position of the same particle and then its speed. He and his colleagues therefore contend that Aharonov and his team’s conclusions were correct in themselves—that the particle moved along one path and the polarization followed the other—but that such differing paths do not apply simultaneously.
As Hofmann’s co-author Jonte Hance, also at Hiroshima University, told New Scientist, “It only looks like (the particle and polarization are) separated because you’re measuring one of the properties in one place and the other property in the other place, but that doesn’t mean that the properties are in one place and the other place, that means that the actual measuring itself is affecting it in such a way that it looks like it’s in one place and the other place.”
A New Way to Catch a Cheshire Cat?
But these critiques are “missing the point,” Popescu says. He agrees that the work and reasoning put forward by Saldanha and Hofmann’s respective groups are correct—but adds that the best way to test any interpretation is to generate testable predictions from each. “As I understand it, there is no direct way to make predictions based on them,” Popescu says in reference to these alternative explanations. “They kind of have a very old-fashioned way of looking at things: there are contradictions, so you stop doing the math.”
With their recent preprint paper, Aharonov and Popescu, together with physicist Daniel Collins of the University of Bristol, have now described how a particle’s spin can move completely independently of the particle itself—without employing a weak measurement. In their new experimental setup, a particle is located in the left half of an elongated two-part cylinder that is sealed at the outer edges. Because of a highly reflective wall in the middle, the particle has a vanishingly small probability of tunneling through to the right-hand side of the cylinder. In their paper, the researchers provide a proof that even if the particle remains in the left-hand area in almost all cases, it should still be possible to measure a transfer of the particle’s spin at the right-hand outer wall. “It’s amazing, isn’t it?” Collins says. “You think the particle has a spin and the spin should stay with the particle. But the spin crosses the box without the particle.”

In a new thought experiment designed to observe the quantum Cheshire cat, physicists would be able to measure the property of a particle in one of two chambers of an elongated cylinder despite the fact that the particle itself would be contained in the other chamber.
This approach would address several of the critical concerns raised thus far. The physicists don’t need weak measurements. Nor do they need to group their experimental results to draw temporal conclusions. (That being said, grouping results would still improve the measurements, given that the angular momentum of the wall itself cannot be determined unambiguously because of the Heisenberg uncertainty principle.) But in this scenario, the only physical principles involved are conservation laws, such as the conservation of energy or the conservation of momentum and angular momentum. Popescu and Collins explain that they hope other groups will implement the experiment to observe the effects in the laboratory.
The new work has piqued Hofmann’s interest. “The scenario is exciting because the interaction between polarization and particle motion produces a particularly strong quantum effect that clearly contradicts the particle picture,” he says.
But he still does not see this as proof of disembodied (particle-free) spin transfer. “For me, this means, above all, that it is wrong to assume a measurement-independent reality,” Hofmann says. Instead quantum mechanics allows a particle’s residence to extend to the right-hand region of the cylinder, even if a residence in the left-hand region seems logically compelling. “I think it is quite clear to Aharonov, Collins and Popescu that the space in front of the wall is not really empty,” he adds.
Saldanha, meanwhile, still sees the researchers as overcomplicating what could be explained as traditional quantum interference effects. When discussing the particle’s very low probability of entering the right-hand side of the experimental setup, he explains, “we have to be careful about a ‘vanishingly small probability’ when we refer to waves.” The wave function of the particle could also expand into the right-hand side of the setup and thus influence the angular momentum of the wall. “The same predictions can be made without such dramatic conclusions,” he says.
In response to these critiques, Popescu says, “This is of course another way of thinking about it. The question is whether this interpretation is useful.” Regardless of which interpretation of the events is correct, the quantum Cheshire cat could enable new technological applications. For example, it could be used to transfer information or energy without moving a physical particle—whether made of matter or light.
For Popescu, however, the fundamental questions of physics play a more important role. “It all started when we thought about how time propagates in quantum mechanics,” he says. “And suddenly we were able to discover something fundamental about the laws of conservation.”
This article originally appeared in Spektrum der Wissenschaft and was reproduced with permission.