September 4, 2024
5 Time required to read
Black hole detectors fulfill Moore’s Law
The famous prediction that microchips will make exponential progress over time could also be applied to unrelated developments, such as the technology used to spot colliding black holes.
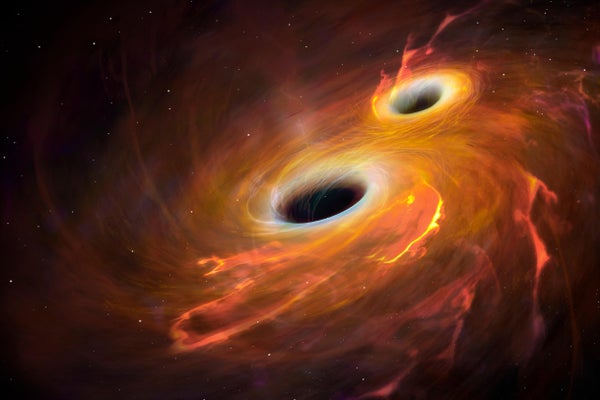
An illustration showing two black holes on the brink of merging, a phenomenon that would generate a massive amount of gravitational waves.
Mark Garlick/Science Photo Library/Alamy Stock Photos
In 1965, engineer and future Intel co-founder Gordon Moore famously noticed that the number of transistors on microchips had been increasing exponentially for several years and predicted that this unstoppable growth would be the driving force behind computing. This astonishing prediction, now known as “Moore’s Law,” has more or less held up ever since.
Given the constant changes and advancements in microchip manufacturing, the consistent trend of exponential growth seems nothing short of miraculous. But in fact, we see this trend in a variety of other areas of technological innovation. One of the most interesting to us (and one that has been largely overlooked so far) is the continued development of a technology called gravitational wave antennas to detect distant black holes.
Black holes form when gravity is too strong for other natural forces to stop the collapse of matter. Black holes are most often born from the death of a massive star with enough matter and density to cause runaway gravitational collapse. And such stellar-mass (or more) black holes survive for a very long time – essentially forever compared to the lifetime of any star. For this reason, black holes exist throughout the universe, with an estimated several million in our galaxy alone.
These strange objects have such an intense gravitational force that they pull in everything that comes near them, even light – hence the name black holes. This is what makes them unique and fascinating, but also makes them difficult to observe. Luckily, we can study them with the help of gravitational waves – ripples that spread throughout space as black holes accelerate. We can detect these waves on Earth using advanced instruments that identify perturbations in space caused by the passing of the waves. These changes are almost inconceivably tiny. For example, when a typical cosmic gravitational wave passes through, the change in the distance between the Sun and Earth (about 150 million kilometers!) is less than the thickness of a human hair.
The more a black hole accelerates, the stronger the gravitational waves it emits, making them easier to detect on Earth. The best way to accelerate a black hole is to collide it with another black hole. These collisions, a game of cosmic billiards, are currently a vital source of information about black holes.
The development of technology to detect black hole collisions began around the 1960s, when Moore made his famous prediction. It is neither easy nor cheap. Detectors have improved over time, but it took more than half a century and more than $1 billion to actually detect a black hole for the first time in 2015. However, since then, rapid improvements in detectors have led to an increasingly rapid rate of new detections, or new discoveries, and it has been reported that about 100 black hole collisions have been confirmed to date.
Just as Moore used the number of transistors in a microchip to quantify technological progress, we propose to use the collision detection rate to quantify progress in black hole detectors. Using this metric, we looked at results from the most sensitive black hole detector, the Laser Interferometer Gravitational-wave Observatory (LIGO), and its planned upgrade, the Cosmic Explorer, to quantify past performance and project future improvements. We measured performance in terms of the actual or predicted number of collisions detected per observing year, from 2000 to 2040 and beyond.
It turns out that from the first discovery in 2015 to some 20 years into the future, the rate of technological progress can be surprisingly well described as exponential growth: the detection rate has doubled roughly every two years. And this trend will likely continue for some time to come, so long as Cosmic Explorer or something equivalent actually gets built and operates. Moore’s Law appears to hold true for black hole detectors as well.
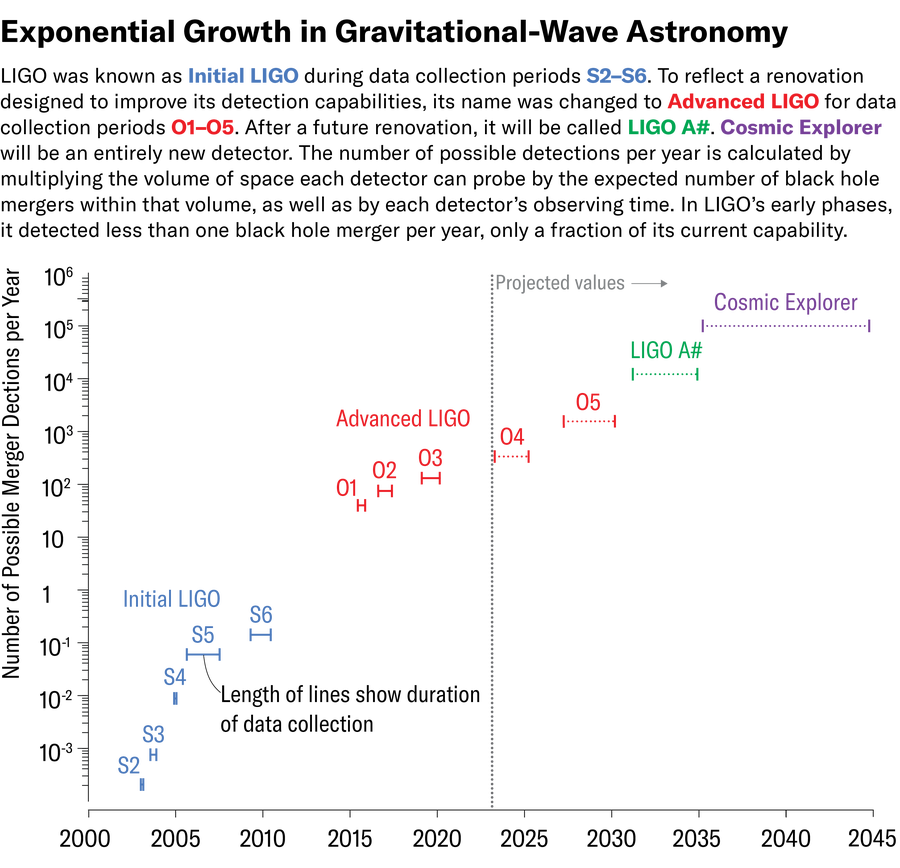
Zane Wolf;Source: National Science Foundation;Szaborcz Malka;Imre Bartos
Improving and operating black hole detectors requires ongoing investment, but this requirement has been essentially met over the past 25 years. It is noteworthy that exponential increases in detector speed have not resulted in significant increases in annual costs. This also means that the cost of detection has dropped significantly. This is another important aspect of Moore’s Law, which predicts that not only will transistor density on microchips increase exponentially, but also with minimal cost increases. In 2015, when black hole collisions were first discovered, the real cost of a black hole collision was about $2 million, but by around 2035, the cost of such detection should be less than $2,000.
Just as improvements in microchips have transformed nearly every aspect of our lives since Moore’s prediction, rapid advances in black hole detection have the potential to transform our understanding of the universe. From groundbreaking insights into the nature of gravity and the life and death of stars, to glimpses into the ultimate fate of the universe, this new and ever-expanding window into the cosmos will undoubtedly change many of our astronomical beliefs.
While it is difficult to quantify the importance of new discoveries, one measure of the importance of black hole collisions to science is the number of scientists directly involved in black hole detectors. Looking at the number of authors on scientific publications from the LIGO detector team (and the collaborating black hole detectors Virgo and KAGRA), we see a five-fold increase between 2004 and 2024.
Supporting science journalism
If you enjoyed this article, please support our award-winning journalism. Subscribe. By purchasing a subscription, you help ensure a future of influential stories about the discoveries and ideas shaping the world today.
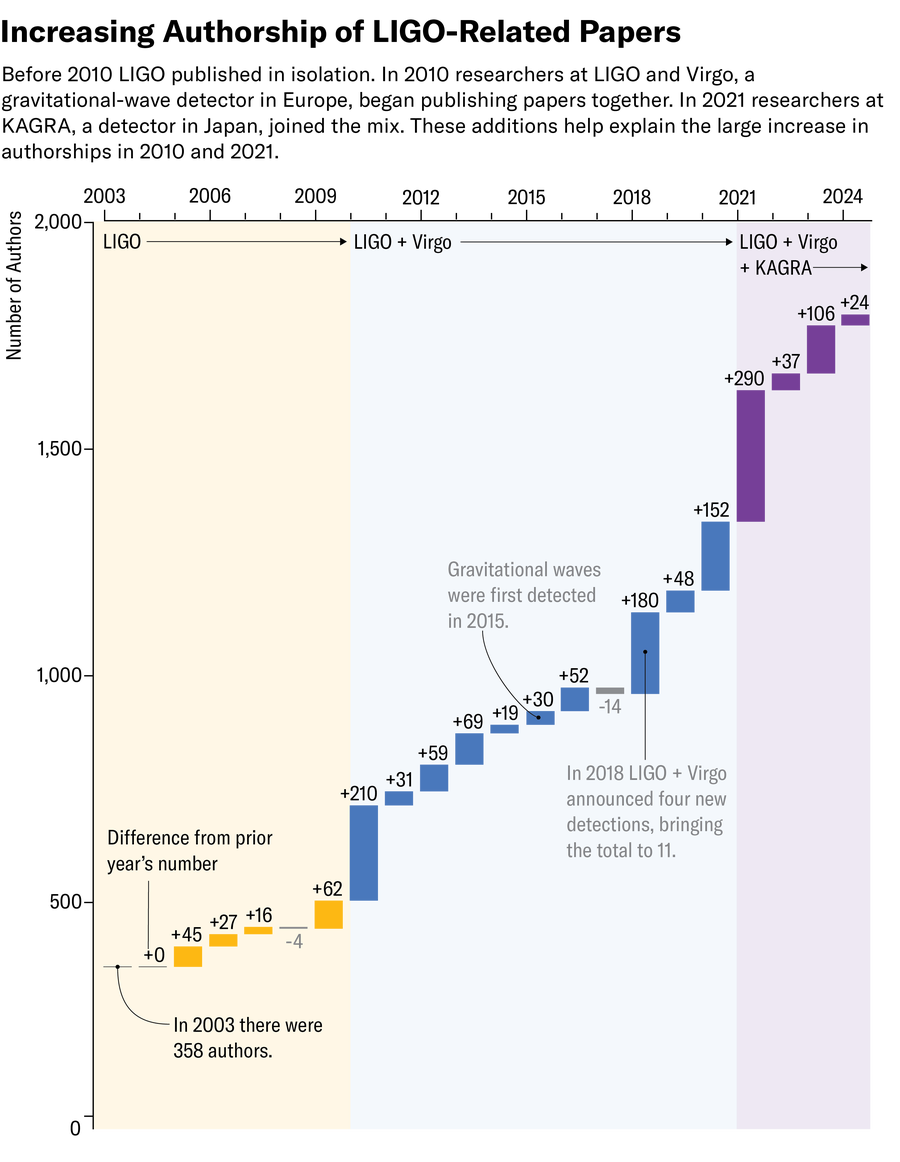
Zane Wolf;Source: National Science Foundation;Szaborcz Malka;Imre Bartos
Although the microchip and the black hole detector rely on very different technologies, their similarity in both improving exponentially over time may hint at more general trends in technology and the nature of human progress. For example, no one is aiming for a constant exponential growth in the sensitivity of black hole detectors, but it may be hard to justify investing money in improving the technology unless the results are significantly better than what already exists.
If exponential growth is indeed a more general technology trend, it may bring benefits beyond those built into the devices we have in our hands today. Setting expectations for the future state of technology, as has been done many times with microchips and computing, can greatly aid in the planning needed to make it a reality. Perhaps, just as exponential technological progress has revolutionized computing and now gravitational wave astronomy, we will see similar radical advances in other domains such as biotechnology and communications in the coming years. Being aware of the promises and perils of these powerful trends may be an important preparation for our brave new future, whatever that may be.
This is an opinion and analysis article and the views of the author are not necessarily those of Scientific American.