New synthesis of superheavy elements suggests long-sought ‘island of stability’
A new way to make superheavy elements could add new rows to the periodic table, allowing scientists to explore uncharted territory in the atom.
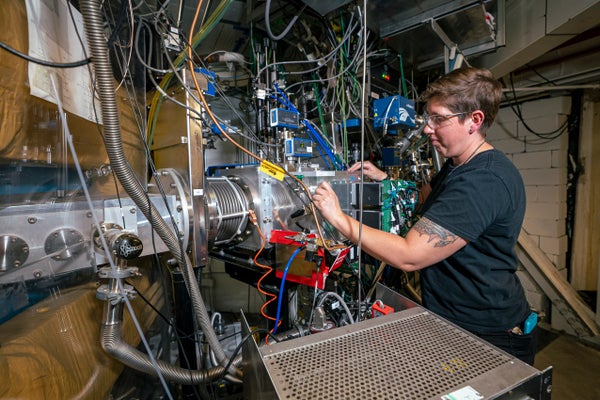
Jacqueline Gates, head of the Heavy Elements Group at the Lawrence Berkeley National Laboratory in California, is leading the effort to create the superheavy element 120.
Marilyn Sargent, Multimedia Productions/University of California, Lawrence Berkeley National Laboratory Board of Trustees
For new, man-made heavy elements on the periodic table, “too big” often means unstable and ephemeral in existence. The more protons and neutrons scientists pack into a “superheavy” nucleus (one with a total number of protons greater than 10), the more fragile the resulting element tends to be. Until now, all man-made superheavy elements could be made to decay almost instantly. But by synthesizing such heavy atoms in a particle accelerator at Lawrence Berkeley National Laboratory, researchers have now taken a big step toward the elusive “island of stability,” a region of the periodic table that is hypothesized to eventually survive long enough for new superheavy elements to buck the trend.
The team was able to fake livermorium, element number 116, using a new method with titanium-50, a rare isotope that makes up about 5 percent of titanium on Earth. By heating the titanium to 3,000 degrees Fahrenheit and channeling it into a high-energy beam, the researchers were able to smash this stream of particles into other atoms to create the superheavy element. While livermorium has been made before using other techniques, this innovative approach could pave the way for the synthesis of new, even heavier elements, expanding the periodic table.
“This result is truly groundbreaking,” says Hiromitsu Haba, a research scientist at the RIKEN Nishina Center for Accelerator-Based Science, who was not involved in the study. Haba adds that the feat is “necessary for the discovery of further new elements.” The study was presented at the Nuclear Structure Conference in July and is currently under review for publication in a journal. Physics Review Letter.
Support science journalism
If you enjoyed this article, please support our award-winning journalism. Subscribe. By purchasing a subscription, you help ensure a future of influential stories about the discoveries and ideas shaping the world today.
The “simple” mathematics of superheavy fusion
Berkeley Lab has an 88-inch cyclotron, a machine that uses electromagnetic fields to nudge atomic nuclei, ejecting some of the surrounding electrons and sending them hurtling toward other, stationary atoms. Using these machines, synthesizing superheavy elements is a simple calculation: to make an element with 116 protons, you need to fuse two nuclei with that total number of protons. But as is often the case in nuclear physics, the process that’s right Very easy.
Traditionally, calcium-48 has been the gold standard isotope for superheavy fusion reactions because of its “doubly magic” property: Atomic nuclei are surrounded by orbital shells of spinning electrons, and nuclei with a “magic” number of protons or neutrons that completely fill the shells are extremely stable. both That’s the exception for particle types. But calcium-48’s low proton count limits its usefulness for creating heavier elements. The heaviest stable element that can be combined with calcium-48 (20 protons) is curium (96 protons), resulting in livermorium (116 protons). Calcium-48 and the heavier berkelium (97 protons) have been used to synthesize element 117, but berkelium is “extremely difficult to make,” says Witold Nazarewicz, principal scientist at Michigan State University’s Rare Isotope Beams Facility, who was not involved in the study. “If you want to make most of the heavier elements, you need a beam with a higher number of protons (than calcium-48).”
To make such a beam, the team turned to titanium-50, which they hoped to fuse with plutonium to create livermorium. “Before we did this, no one knew how easy or hard it would be to make something out of titanium,” emphasizes Jacqueline Gates, leader of Berkeley Lab’s Heavy Elements Group and lead author of the study.
Unlike calcium-48, which is doubly magical and stable, titanium-50 is decidedly non-magical and lacks extreme stability. It also has a melting point nearly twice that of calcium, making it difficult to work with. The low stability of titanium-50 atoms also reduces the probability of successful fusion, even if a collision were to occur. “It’s the difference between seeing a synthesized atom every day versus seeing it every 10 days or less,” Gates explains. Despite these challenges, titanium-50 emerged as the next best candidate because it offered hope that a superheavy element beyond the reach of calcium could be created.
Once the isotopes were prepared and the cyclotron was up and running, the process became a waiting game. Even as a uranium target was continuously bombarded with a titanium beam, the odds of two nuclei colliding were extremely low. “If you swelled up an atom to the size of a football field, the nucleus would be the size of a pea,” Gates says. “We’re bombarding the target with six trillion titanium particles every second, just to increase the statistical chance of getting close to a nucleus.”
Due to the high intensity of the impact and the rarity of successful collisions, it took 22 days to synthesize detectable quantities of the desired livermorium.
In search of an island of stability
The successful use of titanium-50 represents a major step forward in the field of superheavy element synthesis. Not only does the experiment demonstrate the fundamental feasibility of the technique, it also provides important data on the “cross section” associated with titanium-50 particle beams. (Cross section is a measure of the probability that a particular outcome, such as the fusion of livermorium, will occur when two particles collide, based on the energy of the collision.)
Building on this foundation, the next ambitious goal of titanium-50 fusion is the production of element 120, which requires collisions with californium. Element 120 would be the heaviest element ever produced and would be the first in the eighth row of the periodic table. According to some models, the element is also relatively long-lived, making it a bridgehead to the long-sought island of stability. Although theoretical models provide little certainty about the exact energies required to synthesize titanium-based nuclei, these pioneering results provide valuable insights. “(This study) gives us experimental results on cross sections, so we know which (theoretical) models are most reliable,” explains Nazarewicz. Haba adds, “We are looking for nuclei in extreme states, which are still difficult to predict theoretically… but there is absolutely no reason why we cannot synthesize element 120 this way.”
Though the birth of this new element may still be years away, the discovery promises new insights into electron shells and the periodic table, which could have far-reaching implications for atomic physics, materials science and other fields. “You’re accessing g orbitals,” Gates said, referring to a theoretical new electronic configuration that has never been observed before. “It’s like accessing a whole new area of chemistry.”